Disordered materials hold promise for better batteries
February 21, 2014
By Linda Vu
Contact: cscomms@lbl.gov
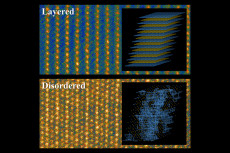
Conventional layered lithium and transition metal cathode material (top) and the new disordered material studied by researchers at MIT (bottom) as seen through a scanning transmission electron microscope. Inset images show diagrams of the different structures in these materials. (In the disordered material, the blue lines show the pathways that allow lithium ions to traverse the material.) Image courtesy of the researchers
Because lithium batteries are among the lightest and most energetic rechargeable batteries available, a lot of research is being done to facilitate their use in electronic devices. Using supercomputers at the National Energy Research Scientific Computing Center (NERSC) and other facilities, researchers at the Massachusetts Institute of Technology (MIT) and Brookhaven National Laboratory (BNL) have found a new avenue for such research: the use of disordered materials, which had generally been considered unsuitable for batteries.
In a rechargeable lithium-based battery, lithium ions—atoms that have given up an electron, and thus carry a net charge—are pulled out of the battery’s cathode during the charging process, and returned to the cathode as power is drained. But these repeated round-trips can cause the electrode material to shrink and expand, leading to cracks and degrading performance over time.
In today’s lithium batteries, cathodes are usually made of an orderly crystalline material, sometimes in a layered structure. When slight deviations from that perfect order are introduced, the battery’s efficiency generally goes down — so disordered materials have mostly been ignored in the search for improved battery materials.
But a recent round of computer models and laboratory experiments show that certain kinds of disorder can provide a significant boost in cathode performance. These surprising findings were published in Science. Authors on the paper include: Jinhyuk Lee, Gerbrand Ceder, Alexander Urban, Xin Li, and Geoffroy Hautier of MIT, and Dong Su of BNL.
Disorder creates new pathways for lithium ions
These days, lithium battery cathodes are striated materials—usually made up of alternating layers of lithium and transition-metal oxides. The materials also typically contain an exact balance of lithium and metal atoms. Until recently, scientists believed that this layering was necessary to provide a clear pathway for lithium to pass in and out of the cathode without bumping into the transition metal oxide layer. After all, high lithium ion mobility is essential for an efficient rechargeable battery. Previously, researchers thought that disorder could significantly reduce mobility.
But it turns out that disordered materials with irregular pathways can still act as efficient channels for lithium mobility—there just needs to be an excess of lithium atoms. Because lithium ions don’t push layers out of shape in disordered materials as the battery charges, this could mean safer and longer lasting batteries.
“In most other lithium materials, as you pull the lithium in and out, it changes dimension, swelling or contracting,” says Ceder. “This swelling and contracting causes all sorts of problems, including fatigue that can lead to cracking.”
But in experiments, a new disordered material—lithium molybdenum chromium oxide—shows a very high dimensional stability. Ceder notes that while the dimensional changes in layered materials can be as much as 5 to 10 percent, in the new disordered material it is only about 0.1 percent — “virtually zero.”
While lithium molybdenum chromium oxide can hold and release significantly more lithium than existing materials, Ceder notes that it produces a lower voltage — meaning its overall performance is about the same as that of existing materials. Despite this revelation, scientists note work is significant because it opens whole new category of possibilities that had previously been ignored in the area of battery research.
Supercomputers: The game changer
According to MIT postdoctoral researcher Alexander Urban, computing played an important role in this discovery. “This work started with a high-throughput screening of a very large compound space, which included the entire periodic table more or less, to identify the best lithium ion cathodes for battery applications,” he says.
To quickly accomplish the high-throughput screening of cathode materials, researchers relied on the Materials Project, co-founded by Ceder. These tools, which are hosted on a Science Gateway at NERSC, aims to take the guesswork out of finding the best material for a job—be it a new battery electrode or a lightweight spacecraft body—by making the characteristics of every inorganic compound available to any interested scientist. The project’s ultimate goal is to cut the amount of time it takes to bring new materials to market by half. It currently takes about 18 years to move materials from R&D to market.
“Once we had a list of candidates, Jinhyuk Lee began synthesizing compounds and found that molybdenum chromium oxide worked very well as a cathode,” says Urban. “But surprisingly, the material did not have a traditional layered look—it was disordered. This is when computation came back into play, now we had to figure out why this structure worked so well.”
To solve this mystery, Urban used NERSC’s Hopper to compute lithium migration barriers in a number of different disordered structures and diffusion channels. “There are many different possibilities for how lithium ions can migrate from one side of the crystal structure to the other,” he says.
The calculations showed that active diffusion channels in disordered materials are different than those in traditional materials. Unlike traditional cathodes, which have an exact balance of transition metals and lithium ions, the disordered materials require an excess of lithium to be effective. The computer runs also helped researchers determine the concentration of lithium excess required to produce an effective battery.
“Our analysis shows a new direction that we can take in searching for even better materials for batteries,” says Urban. “It is also a great example of the extraordinary science that can be accomplished when simulations (theory) computed in real-time feed into laboratory experiments and vice versa.”
This research was funded by the Robert Bosch Corporation, Unicore Specialty Optics and Chemicals, Samsung, and the U.S. Department of Energy.
This story was adapted from an MIT press release.
More information Berkeley Lab’s contribution to the Materials Project.
About Computing Sciences at Berkeley Lab
High performance computing plays a critical role in scientific discovery. Researchers increasingly rely on advances in computer science, mathematics, computational science, data science, and large-scale computing and networking to increase our understanding of ourselves, our planet, and our universe. Berkeley Lab’s Computing Sciences Area researches, develops, and deploys new foundations, tools, and technologies to meet these needs and to advance research across a broad range of scientific disciplines.