Mapping the March to Methodical Materials
NERSC Helps Corroborate Two Distinct Mechanisms in Ferroelectric Material
September 18, 2014
Contact: Kristin Manke, kristin.manke@pnnl.gov, +1 509.372.6011
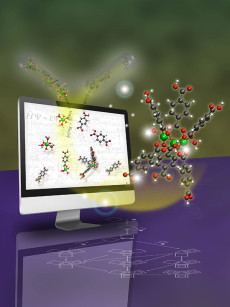
Scientists from Pacific Northwest National Laboratory demonstrated the fundamental reactions that occur when synthesizing the building blocks of a metal-organic framework. Image: PNNL
Selective and reactive, metal-organic frameworks (MOFs) could replace inefficient materials in batteries, catalysts, solid-state heat pumps, and other products where gas separation and storage are vital. While scientists knew how to synthesize MOFs, they didn't know the details of the reactive steps that occurred during assembly of the framework.
Now, a trio of scientists at DOE’s Pacific Northwest National Laboratory (PNNL) determined the individual reactions and the energy needed at each step to form the basic unit of a popular MOF, MIL-101.
The team took a computation- and simulation-based approach that allowed them to delve into the intricacies of the reaction sequence that creates the short-lived intermediate molecules that form the MOF building blocks. Understanding those steps is vital to eventually being able to synthesize the materials by the boxcar, not the test tube.
“If you want control over a process, you need to know what's going on and when; in other words, you need to know the mechanism,” said Vassiliki-Alexandr Glezakou, a computational chemist at PNNL and the corresponding author on the study, which was published September 18, 2014 in Chemistry of Materials.
Glezakou was also the study’s principal investigator at the National Energy Research Scientific Computing Center (NERSC), where some of the computations were run.
The Power of MOFs
Successfully tailoring MOFs could lead to catalysts that quickly produce fuels, chemicals and other products with less waste and requiring less energy. They could lead to batteries that let electric cars travel greater distances between charges. They could revolutionize technologies as diverse as sensors and building chillers. To do so, the MOFs designed in laboratories must be made on an industrial scale at low cost. The study, funded by DOE's Office of Fossil Energy, describes how MOF's building blocks are formed. Knowing the fundamental steps gives scientists insights into the speed, energy, and efficiency of MOF nucleation.
“To get smarter about how to scale up synthesis of MOFs so that they can be deployed in commercial products, we need a much better understanding of the basics of the self-assembly process than we have today,” said B. Peter McGrail, a Laboratory Fellow at PNNL who is leading several projects developing MOFs for gas separations and building/residential cooling.
Basic Building Blocks
To understand how a MOF is formed, the team began by looking at the basic building block, known as a secondary building unit (SBU). The SBU contains metal atoms and linkers or strands of carbon-based molecules. Connect SBUs together, create a MOF.
“Forming the SBUs is the rate-limiting step in creating different MOFs," said David Cantu, a chemical engineer who worked on this study as part of his postdoctoral fellowship at PNNL. "The faster you can make SBUs, the faster you can make a MOF."
Using resources at EMSL, NERSC and PNNL Institutional Computing, the team examined the reactivity of the material's basic components. "We looked at these initial reactions using computational chemistry to examine very specific scenarios that give us proof of what's happening and let us know how to influence the outcomes," said Glezakou.
The team worked with MIL-101, a chromium-based MOF, because of the availability of experimental data. The MIL-101 SBU has a single oxygen atom, surrounded by a triad of chromium ions and wrapped up by six carboxylic linker molecules. The reactions were not quite what the team expected. They discovered a very specific order in which the chromium ions and organic linkers are combined.
Initially, each of the three chromium atoms connects to a single linker molecule. Then, two of the chromium-linker sets combine. This is the most energy-intensive step along the most efficient path. Next, the two-metal linker unit and a third chromium-linker unit merge together. Water molecules constantly vie for a place close to the chromium atoms, blocking access to the metal. Working around the water molecules takes energy, but becomes easier as the unit grows. Finally, the other three linker molecules attach.
“In some ways, this study was a lot like writing,” said Cantu. “We started with a blank page. We knew the introduction and the conclusion, but not what happened in between. We figured out what happened in between.”
Next, the team is looking to scale up their research to determine the steps involved in stacking up the basic units to form MOFs.
“We saw how the SBU is formed,” said Cantu. “Now we want to see how the whole material is formed.”
About Computing Sciences at Berkeley Lab
High performance computing plays a critical role in scientific discovery. Researchers increasingly rely on advances in computer science, mathematics, computational science, data science, and large-scale computing and networking to increase our understanding of ourselves, our planet, and our universe. Berkeley Lab’s Computing Sciences Area researches, develops, and deploys new foundations, tools, and technologies to meet these needs and to advance research across a broad range of scientific disciplines.