Taming Plasma Fusion Snakes
Supercomputer simulations move fusion energy closer to reality
January 24, 2014
Kathy Kincade, +1 510 495 2124, kkincade@lbl.gov
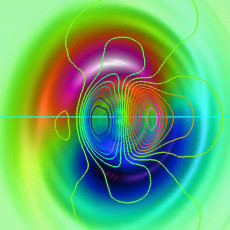
Researchers have been able to see and measure plasma snakes – corkscrew-shaped concentrations of plasma density in the center of a fusion plasma -- for years. 3D nonlinear plasma simulations conducted at NERSC are providing new insights into the formation and stability of these structures. Image credit: Linda Sugiyama
Controlled nuclear fusion has held the promise of a safe, clean, sustainable energy resource for decades. Now, with concerns over global climate change growing, the ability to produce a reliable carbon-free energy source has taken on new urgency.
Fifty years of nuclear fusion research has brought many advances, but technical hurdles remain. Fortunately, supercomputing resources at Department of Energy’s National Energy Research Scientific Computing center (NERSC) are helping researchers address these issues and move fusion energy closer to reality.
In a fusion reaction, energy is released when two hydrogen isotopes (tritium and deuterium) are fused together to form a heavier nucleus, helium. To achieve high enough reaction rates to make fusion a useful energy source, the hydrogen gas must be heated to extremely high temperatures -- more than 100 million degrees Celsius — which transforms it into a hot gas known as plasma.
Unfortunately, the high energy required to bring the plasma to these temperatures also drives the formation of plasma instabilities that need to be controlled in order to hold the plasma together and allow fusion to take place.
One commonly observed instability is the plasma density snake, named for its corkscrew-shaped appearance. Impurity snakes have been a regular feature in every major tokamak fusion experiment of the last 25 years, according to Linda Sugiyama, a principal research scientist in the Laboratory for Nuclear Science at the Massachusetts Institute of Technology (MIT) and a long-time NERSC user. But only recently have Sugiyama and colleagues been able to explain the formation and stability of these snakes, which could lead to insights into how to control them and help maximize the safety and performance of next-generation reactors such as ITER, an international tokamak facility under construction in France.

A measured time sequence of a plasma snake (side and edge views).
“Snakes themselves are not too bad since they mainly just sit in the plasma,” Sugiyama explained. “But they’re closely related to other helical instabilities that occur in the same place at the same time that can cause the central temperature and density of the plasma to suddenly drop—what we call a ‘sawtooth crash,’ which is very bad for the fusion reaction rate. The plasma recovers and reheats, but the periodic drops aren’t ideal for fusion.”
In addition, if the area where the crash occurs is large enough, it can trigger other instabilities further out in the plasma that can cause it to self-destruct. This could cause significant damage in burning plasmas such as ITER because there is a lot of energy in the plasma that suddenly hits the walls, she added.
A New Kind of Snake
A tokamak is a type of fusion reactor considered by many to be the best candidate for producing controlled thermonuclear fusion power. It uses toroidal (doughnut-shaped) vessels and extremely strong magnetic fields to confine the plasma as it is heated up. Unfortunately, snakes can trap impurities released from the plasma-facing walls of the tokamaks, and these impurities radiate large amounts of energy that cool the plasma and undermine its ability to create a fusion reaction.
“When you try to heat up magnetically confined plasma, the temperature in the center builds up,” Sugiyama explained. “While this is good for fusion reaction, if it reaches a certain point it can trigger various 3D magnetohydrodynamic (MHD) instabilities [such as snakes], which are the fastest growing and most dangerous.”
To date, the most commonly studied snakes have been those induced by the injection of deuterium pellets into the plasma. But novel spectroscopic imaging diagnostics have enabled researchers to identify a new type of snake with unprecedented resolution: the impurity snake, which is produced by an accumulation of impurity ions rather than deuterium ions from injected fueling pellets.
“Frozen solid pellets are usually shot into the plasma and penetrate far before ionizing and becoming plasma, so impurities fall into the edge of the plasma and have to diffuse inward more slowly,” Sugiyama noted. “Here, impurity snakes are important because they’re the kind that were measured experimentally in Alcator C-Mod, which doesn’t have a pellet injector.”
In research published in Physical Review Letters and Physics of Plasma, Sugiyama and her MIT collaborators explained how computer simulations demonstrated the existence of impurity snakes and yielded new insights into the formation and stability of these snakes. Nonlinear MHD simulations using the extended MHD code M3D also helped them better understand the complex relationship between impurity snakes and another type of instability of the plasma center known as sawteeth.
“Because the mechanism for forming these snakes was so different from the assumptions that had been made previously, we did simulations of the snakes to show that it was possible for them to form and be self-sustaining in a way that was seen in the experiment,” Sugiyama explained.
Plasma Pressure, Density and Temperature
MHD models have typically focused on the plasma pressure only. But pressure is a product of density and temperature and the snake is a density concentration. When Sugiyama and her colleagues added density to the model, they found that the formation of the snakes cannot be explained by plasma pressure alone, as previously thought. Rather, the formation of the snakes is a result of the relationship between the evolving plasma density and temperature that produces a particular plasma pressure. Unlike the density, the pressure showed very little peaking.
“What made these simulations different was that we did both temperature and density evolution; normally people only do pressure,” Sugiyama explained. “But you really need to know the plasma density separate from the temperature to understand the snakes. We had no really good simulation of snakes prior to this, and this is where the NERSC computers come in.”
The codes she and her colleagues have developed and run on the Edison and Hopper supercomputers at NERSC are capable of rapidly running and re-running hundreds or thousands of virtual experiments on plasma behavior in tokamaks in the time it would take to construct just one physical experiment, and at a fraction of the cost and with far more detailed information about the results, she emphasized.
Fusion research has long been dependent on computer simulations, and NERSC was established 40 years ago to support such research. Even with new experimental tokamaks coming on line, fusion is expected to be heavily dependent on numerical simulations using well-verified codes and models, since experimental time on large experiments such as ITER will be limited and valuable. Even before operation begins, simulations can provide insights into the different modes of operation that are possible with the device and will enhance the development of optimization and control strategies.
"Using these codes, we have already learned much about how to avoid or control various instabilities in order to maximize the performance of a future reactor, and we expect to continue to improve," Sugiyama concluded.
About Computing Sciences at Berkeley Lab
High performance computing plays a critical role in scientific discovery. Researchers increasingly rely on advances in computer science, mathematics, computational science, data science, and large-scale computing and networking to increase our understanding of ourselves, our planet, and our universe. Berkeley Lab’s Computing Sciences Area researches, develops, and deploys new foundations, tools, and technologies to meet these needs and to advance research across a broad range of scientific disciplines.