Supercomputers Help Identify Efficiency-Limiting Defects in LEDs
July 22, 2016
Contact: Kathy Kincade, kkincade@lbl.gov, +1 510 495 2124
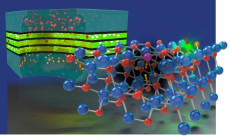
A conceptual illustration of how defects in a crystal lattice might contribute to nonradiative recombination of electrons and holes in LEDs. Image: Peter Allen
Light-emitting diodes (LEDs) have found firm footing in the lighting industry, not just for blue and green light emission but also as white light emitters for general lighting applications. Even so, many of the materials used in these devices suffer from inherent material defects that can lead to less-efficient solid-state lighting.
In LEDs, light emission occurs when electrons and holes recombine radiatively. But occasionally, material defects cause them to not emit light when recombining. This nonradiative recombination results in a loss of efficiency of the LED. While this phenomenon, known as the Shockley-Read-Hall (SRH) recombination, has been known for over 60 years, the microscopic origin of SRH in III-nitride semiconductors was unknown.
Now a team of researchers at the University of California, Santa Barbara (UCSB) believe they have made new strides in achieving more energy-efficient LEDs by proposing a microscopic mechanism for SRH and identifying specific defects that can explain the efficiency reduction observed experimentally in LEDs. Their research, which utilized 500,000 hours on NERSC supercomputers, was featured in the April 4, 2016 Applied Physics Letters and on the journal cover.
“We wanted to identify which defects could be responsible for the efficiency loss that has been observed in LEDs, and that takes a lot of computer time because we needed to screen a lot of defects to be able to identify which ones were most likely candidates for such loss,” Van de Walle said. “So a lot of individual defects need to be looked at. And on top of that, for the defects that look plausible, it is a lot of work to actually calculate these nonradiative recombination rates.”
This study was enabled by the development of a theoretical formalism necessary to calculate the rate at which defects capture electrons and holes, an effort in which one of the authors of the present work, Audrius Alkauskas, played a key role. That theoretical formalism was described in detail in a Physical Review B paper published in 2014. The approach involves the use of first-principles methods based on density functional theory (DFT), including advanced techniques based on hybrid functionals.
“It was the combination of the intuition that we have developed over many years of studying point defects with these new theoretical capabilities that enabled this breakthrough,” Van de Walle said.
Gallium Vacancies with Oxygen, Hydrogen
The defects that were identified involve complexes of gallium vacancies with oxygen and hydrogen. These defects had been previously observed in nitride semiconductors, but until now their detrimental effects were not understood, explained Cyrus Dreyer, who performed many of the calculations and is the lead author on the paper.
“Having access to NERSC resources is absolutely essential for being able to do this work because we are keenly interested in continuing this research,” Van de Walle said. “The results we reported in Applied Physics Letters are very interesting and will hopefully have high impact, but we are not claiming these defects are the only ones that play a role. We are actively pursuing other defects and impurities that we suspect are also important for the efficiency loss.”
The work points the way toward fabrication of more efficient devices, a central goal for solid-state lighting and an array of other electronic devices, he added.
“With this research we are addressing a problem that has been around for almost 70 years,” Van de Walle said. “The nonradiative recombination process has been well documented and is not limited to LEDs—it happens in all kinds of optoelectronic and electronic devices, including transistors. Over the years, people have reported experimental observations of the phenomenon and have attempted to model it using empirical modeling, but they were never successful. Our work is really the first time a model, backed up by heavy-duty computer calculations, has been able to reliably predict what specific defects are doing.”
About Computing Sciences at Berkeley Lab
High performance computing plays a critical role in scientific discovery. Researchers increasingly rely on advances in computer science, mathematics, computational science, data science, and large-scale computing and networking to increase our understanding of ourselves, our planet, and our universe. Berkeley Lab’s Computing Sciences Area researches, develops, and deploys new foundations, tools, and technologies to meet these needs and to advance research across a broad range of scientific disciplines.