Boosting the Next Wave of Accelerators
New Technique Speeds Simulations by up to a Million-fold
March 17, 2011
Contact: Paul Preuss, paul_preuss@lbl.gov, 510.486.6249
NERSC Contact: Margie Wylie, mwylie@lbl.gov, , 510.486.7421
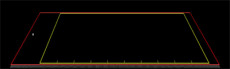
Simulations of a laser-plasma wakefield are shown in a standard “laboratory frame,” stationary observer and moving beam. The laser pulse is represented in blue and red; the wakefields are colored pale blue and yellow. The shining dot represents the electron beam. (Images and animations by Jean Luc-Vay using a visualization package developed at Berkeley Lab by David Grote and Vay based on the open-source Opendx package)
Albert Einstein’s most famous thought experiment is proving its worth once again as researchers use it to help speed up the modeling (and thus design) of so-called “tabletop” accelerators.
Particle accelerators, such as CERN’s Large Hadron Collider (LHC), help physicists unlock the fundamental secrets of matter and the beginnings of our universe. But conventional accelerators are large and expensive. An emerging new class of compact accelerators is being designed to cost less and pack more power into much smaller spaces than today’s machines.
However, generating computer models of tabletop accelerators has proven difficult, slow, and costly. To solve this problem, a team of scientists computing at the National Energy Research Scientific Computing Center (NERSC) has perfected a new method that generates models anywhere from 10,000 to a million times faster than before.
This “boosted frame” approach uses a feature of special relativity to calculate in a just a few hours models that once took days, or even weeks, to complete. The method even allows researchers to execute models that weren’t previously within the realm of possibility.
“For the first time we can vary parameters and get results in a matter of days at full physics, at full scale. This was totally unthinkable before,” says Jean-Luc Vay of the Accelerator and Fusion Research Division (AFRD) at Lawrence Berkeley National Laboratory (Berkeley Lab). Vay, who led the team, first proposed the boosted-frame approach in 2007. He and colleagues Cameron Geddes of the LOASIS Program in AFRD, Estelle Cormier-Michel of the Tech-X Corporation in Denver, and David Grote of Lawrence Livermore National Laboratory published their findings in the March 2011 issue of the journal Physics of Plasma Letters.[1]
New Kind of Accelerator, New Kind of Model
The LHC uses giant, supercooled magnets to sling atomic particles, such as electrons, around a 27 km underground tunnel. When the particle beams reach nearly the speed of light they are steered into head-on collisions. Scientists study the wreckage or “decay” of these smash-ups to learn more about the nature of matter.
However, to reach higher speeds (or “energies” as scientists term it), conventional magnetic accelerators, such as the LHC, must grow ever larger. The best hope for the high-energy machines of the future may lie in smaller, more powerful and less expensive laser-plasma accelerators. BELLA (the Berkeley Lab Laser Accelerator) is one of them.
Now being built by the LOASIS program at Berkeley Lab, BELLA is remarkably compact. In just one meter, BELLA will accelerate an electron beam to 10 billion electron volts, one-fifth the energy achieved by the long linear accelerator at the SLAC National Accelerator Laboratory.
Laser-plasma accelerators like BELLA work by sending a very short laser pulse through a plasma measuring a few centimeters or more. In its wake, like a speedboat on water, the laser pulse creates waves in the plasma. These alternating waves of positively and negatively charged particles set up intense electric fields. Bunches of free electrons “surf” this wakefield and are accelerated to high energies.
But realizing the promise of laser-plasma accelerators crucially depends on being able to simulate their operation in three-dimensional detail. Until now such simulations have challenged or exceeded even the capabilities of supercomputers.
“The most common way to model a laser-plasma accelerator in a computer is by representing the electromagnetic fields as values on a grid, and the plasma as particles that interact with the fields,” explains Geddes, a member of the LOASIS scientific staff who has long worked on laser-plasma acceleration. “Since you have to resolve the finest structures — the laser wavelength, the electron bunch — over the relatively enormous length of the plasma, you need a grid with hundreds of millions of cells and time steps.”
As a result, while much of the important physics of BELLA is three-dimensional, direct 3-D simulation was initially impractical. Just a one-dimensional simulation of BELLA required 5,000 hours of supercomputer processor time on NERSC’s Franklin supercomputer.
Using their boosted-frame method, Vay’s team has achieved full 3-D simulations of a BELLA “stage” (one length of plasma) in just a few hours of supercomputer time, calculations that would have been beyond the state of the art just two years ago.
Not only are the recent BELLA calculations tens of thousands of times faster than conventional methods, but they also overcome problems that plagued previous attempts to implement the boosted-frame idea.
A 'Boosted' Point of View
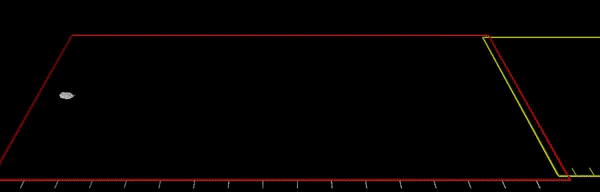
This image shows the “boosted frame,” in which the observer moves at near light speed. The laser pulse is represented in blue and red; the wakefields are colored pale blue and yellow. In this frame, the plasma (yellow box) has contracted and the wave fronts are fewer and farther apart, resulting in far fewer calculations and faster results.
If the team could figure out how to boost their observation point to near light speed, the length of the plasma would dramatically shorten, the wave would lengthen, and the time it takes to cross the plasma would shorten. That would mean far fewer calculations and less computer time to achieve the same results.
Sounds simple, but boosting the simulation’s viewpoint turned out to be fraught with difficulties. Early attempts to apply the boosted-frame method to laser-plasma wakefield simulations were limited by numerical instabilities, small errors that are magnified the longer a calculation runs. Calculations could still be speeded up tens or even hundreds of times, but that was only a tiny fraction of the full promise of the method.
Vay’s team showed that using a particular boosted frame, that of the wakefield itself, gave them near-optimal calculation speeds. Also, calculating fewer oscillations made it easier to deal with numerical instabilities. Combining these advantages with special techniques for interpreting the data between frames, Vay and team were able to exploit the full potential of the boosted-frame principle.
“We produced the first full multidimensional simulation of the 10 billion-electron-volt design for BELLA,” says Vay. “We even ran simulations all the way up to a trillion electron volts, which establishes our ability to model the behavior of laser-plasma accelerator stages at varying energies. With this calculation we achieved the theoretical maximum speed-up of the boosted-frame method for such systems — a million times faster than similar calculations in the laboratory [stationary observer] frame.”
Speedier calculations will allow scientists to simulate the detailed physics they need in order to develop laser plasma accelerators not only for future particle colliders, but for other applications, as well.
Vay used 240,000 processor hours on NERSC’s Franklin, a Cray XT4 with 38,000 processor cores, for the team’s initial simulations. The team plans to take advantage of their new boosted-frame method together with new supercomputers like NERSC’s Hopper, a 150,000-core Cray XE6, to produce high-resolution simulations that can accurately resolve very high quality beams.
This story was adapted from a news release written by Paul Preuss.
About Computing Sciences at Berkeley Lab
High performance computing plays a critical role in scientific discovery. Researchers increasingly rely on advances in computer science, mathematics, computational science, data science, and large-scale computing and networking to increase our understanding of ourselves, our planet, and our universe. Berkeley Lab’s Computing Sciences Area researches, develops, and deploys new foundations, tools, and technologies to meet these needs and to advance research across a broad range of scientific disciplines.